We Knew That Already
- By Koji Mukai
- March 17, 2016
- Comments Off on We Knew That Already
The Advanced LIGO group announced the first direct detection of gravitational waves on February 11, 2016 – it was a momentous day for physics and astrophysics. But the way some news outlets have reported it, saying things like “this proves Einstein was right,” sounds a little bit off to me: we knew that already. The prior proof of the existence of gravitational waves was strong enough to earn a Nobel Prize in Physics in 1993. Admittedly, this was an indirect proof, which is different from a direct detection – and I certainly don’t mean to downplay the importance of the Advanced LIGO result.
Russell A. Hulse and Joseph H. Taylor discovered the binary pulsar in 1974, which eventually won them the Nobel. This is by far the best astrophysical evidence for gravitational waves until today – but there was at least one line of evidence found earlier, albeit far less conclusive. In the study of cataclysmic variables (CVs for short), the research field I work on, the first hint was published in a classic 1962 paper by Robert P. Kraft, Jon Mathews and Jesse L. Greenstein, titled “Binary stars among cataclysmic variables. II. Nova WZ Sagittae: a possible radiator of gravitational waves.”
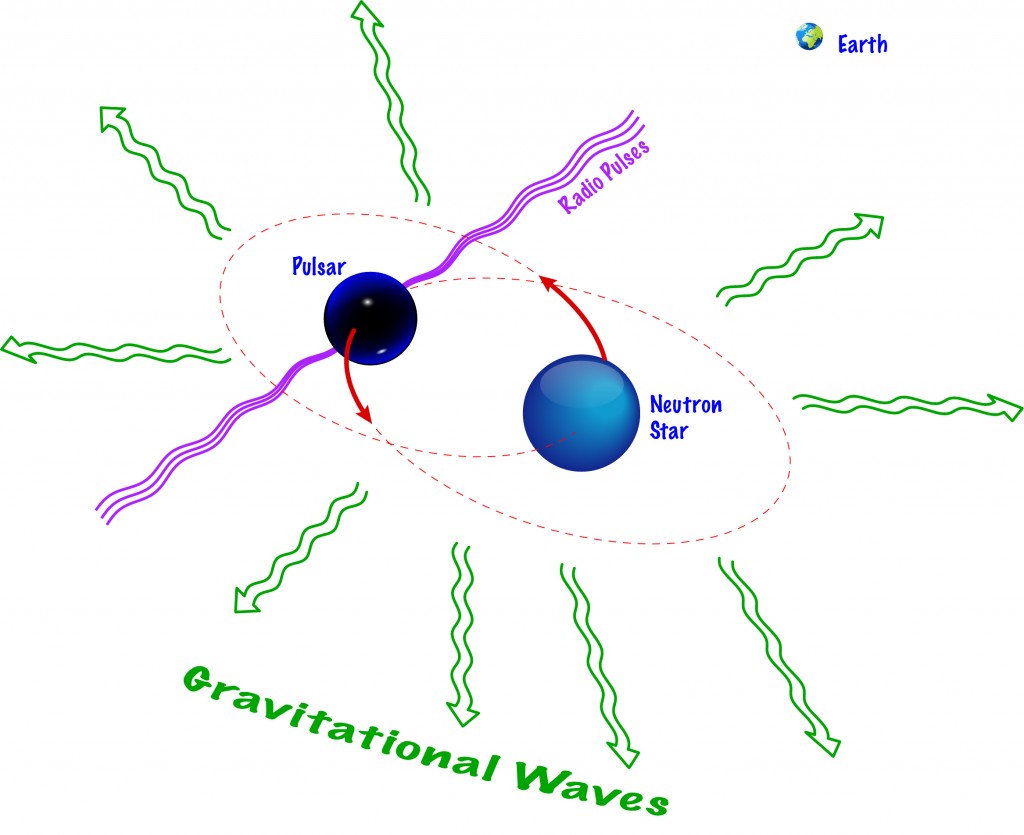
Diagram of Hulse/Taylor’s Nobel Prize winning binary system. The system has a neutron star that orbits with a pulsar – the pulsar is a neutron star that sweeps a strong radio beam toward the Earth as it rotates. As they orbit, they emit gravitational waves, causing the orbit to shrink. Credit: Shane L. Larson
CVs are compact binary systems, in which a white dwarf accretes matter from a red dwarf companion star. A white dwarf is the nuclear ash of a low mass star like the sun at the end of its life – with, ~ 80% of the mass of the Sun but only as big as the Earth. The entire binary system is only about the size of the Earth-Moon system. In any binary system, motions of other objects (planets etc.) are determined by the combined gravity of two stars, and the centrifugal force. If you are very close to one of the stars, its gravity would be dominant and objects fall toward, or orbit around, it. Such regions are called Roche lobes.
A white dwarf is so small it’s well within its Roche lobe; a red dwarf can fill its Roche lobe, or even overfill. When that happens, matter spills over from the red dwarf to the white dwarf, leading to accretion onto the white dwarf. This is the defining characteristic of CVs. Accretion liberates a large amount of potential energy, since the white dwarf is very dense and has a strong gravitational field. The light we observe from CVs is mostly due to accretion, and not from the white dwarf and the red dwarf themselves, which are both dim as stars go.
In this movie, the binary system consists of a main sequence star like our sun, and a neutron star 1.4 times more massive than the sun. As the star evolves, it expands and begins transferring mass to the neutron star. Once it expends all of its nuclear fuel, it collapses into a white dwarf. Credit:scienceinsociety
The thing is, accretion in such binary system is not self-sustaining. Roche-lobe overflow should enlarge the binary orbit, make the Roche lobe around the red dwarf larger, and hence stop further accretion. Yet we know accretion is continuing in CVs: something is forcing continued Roche-lobe overflow, either by enlarging the red dwarf (unlikely) or by shrinking the orbit. Kraft and co. proposed that gravitational wave radiation was the culprit. Their calculations back it up: Einstein’s predictions of how much the orbit of WZ Sagittae should shrink every year because of gravitational waves appear to drive the right amount of mass accretion in WZ Sagittae, whose orbital period is only 80 minutes. The compactness of this binary makes it a stronger source of gravitational waves than in longer period CVs.
With further studies of CVs by many observers in mind, John Faulkner published a paper in 1971 with more calculations, firmly establishing gravitational wave radiation as the driver of accretion in CVs. By the early 1980s, the theory has progressed to the point of explaining the orbital period distribution of CVs (e.g., the paper by Bohdan Paczynski and Ryszard Sienkiewicz published in 1983). I personally think that these results on CVs were strong enough evidence for the existence of gravitational waves to win a civil case in the US court system, for which only a preponderance of evidence is required. It didn’t rise to the level of “proof beyond any reasonable doubt,” however. This is because accretion in CVs is messy, which made our inferences about gravitational wave radiation using CVs rather imprecise, with the potential for some clever theorists to devise alternative explanations.
The pulsar that Hulse and Taylor discovered in 1974 is a very precise clock, from which we see a pulse of radio waves once every 60 milliseconds or so. But it’s also in a binary system, with an orbital period of 7.75 hours: the distance from the Earth to the pulsar changes periodically, every 7.75 hours, with an amplitude of about 900,000 km, a distance light takes only 3 seconds to travel. So the arrival time of the radio pulses, emitted with a strict period but with a varying travel time, is modulated by 3 seconds every 7.75 hours. This allowed radio astronomers to measure the orbital period, and any changes in the orbital period, of the binary pulsar very accurately. Over the years, the period is measured to be becoming shorter, exactly as much as it should due to gravitational wave radiation. Einstein was right. This is a clean test of relativity, strong enough to win a criminal case.
In fact, I would go one step further. Normal, competent scientists would have inferred the presence of something like the gravitational waves, if they had the same observations of the binary pulsar but not Einstein’s theory of gravitational waves. No genius necessary. Observations made it clear that something was taking energy and angular momentum away from the binary pulsar. The empirically derived theory of this mystery drain would have matched the theory of gravitational waves derived from general relativity.
This imaginary alternative history of how gravitational waves could have been discovered, actually matches the history of the neutrino. The neutrino was proposed by Wolfgang Pauli in 1930 because, without something like it, energy, momentum, and angular momentum appeared not to be conserved during beta decays. But neutrinos are elusive particles that could go through a block of lead one light year long without being stopped. Neutrinos were only experimentally confirmed in 1956. (Frederick Reines was awarded a Nobel prize in 1995 for this pioneering discovery.) It took 30 further years before we had the first detection of neutrinos from outside the solar system, the supernova 1987A in the Large Magellanic Cloud.
Maybe the advanced LIGO result puts us in a similar position to the one we had regarding neutrino astronomy back in 1987. Even the detection of a single gravitational wave event is a Nobel-worthy feat of scientific and technical breakthrough. However, the true astrophysical significance will only be achieved if we can continue the progress to the point of routinely detecting more and more gravitational wave sources.
Coming back to CVs, there is a subclass called AM CVn type stars (some might consider it a cousin, rather than a subset, of CVs). They have even shorter orbital periods than WZ Sagittae (as short as 5.4 min), and many are thought to be white dwarf-white dwarf pairs. Because they are so compact, they are even stronger sources of gravitational waves than normal CVs. However, the gravitational waves they emit are of the wrong frequencies for the Advanced LIGO. It will take a spaced-based gravitational wave observatory, such as eLISA currently being proposed, to detect gravitational waves from AM CVn type stars. As with LIGO, eLISA is a technically extremely challenging endeavor: so much so that ESA, the European Space Agency, just launched the LISA pathfinder mission to see how well the current technology works, to see if we are ready to start work on eLISA in the very near future.
I don’t think this history makes the tremendous achievement of the Advanced LIGO team any less spectacular. Now that you have read this far, maybe you can decide for yourself which headlines were more accurate. And let’s hope for future headlines from Advanced LIGO, LISA pathfinder, and beyond.